Agenda
PhD Thesis Defence
- Tuesday, 31 January 2023
- 15:00-16:30
- Aula Senaatszaal
Ultrasound Imaging through Aberrating Layers
Pim van der Meulen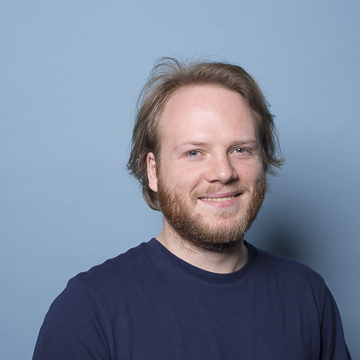
Whereas aberrating layers are typically viewed as forming an impediment to medical ultrasound imaging, they can surprisingly also be used to our benefit. As long as we can model the effect of an aberrating layer,we can utilize ‘model-based imaging’, the imaging technique explored throughout this thesis, to reconstruct ultrasound images where traditional beamforming methodswould fail, employing the ever increasing computational power available to us nowadays. Not only does this allow us to image through layers, but it also leads to interesting applications, such as 3D ultrasound imaging with spatially undersampled data, using an aberrating ‘coding mask’. The formulation of a measurement model, a fundamental part of model-based imaging, also gives insight into the imaging problem mathematically, and allows us to investigate methods for estimating the effect of an aberrating layer ‘blindly’, i.e., without explicitly measuring it.
In this thesis, we thus investigate (a), imaging through a layer when the layer’s aberration effect is known, and how it can be applied to imaging with spatially undersampled data, and (b), methods and algorithms for estimating the effect of the aberrating layer without knowing it a priori.
In the first part of this thesis, we illustrate how using model-based imaging can be utilized for 3D ultrasound imaging using a single ultrasound transducer, and equipping it with a plastic coding mask. The plastic mask acts as an analog coder, that scrambles the transmitted and received waves in a manner that is location dependent. As a result, the temporal shape of an ultrasound echo can be used instead of the traditional method of using phase differences between sensors in a sensor array. Imaging is instead accomplished using model-based imaging. By measuring the pulse-echo response of each pixel, we can form an image by solving a regularized linear least squares problem, which takes into account the measured pixel-specific pulse-echo signals. The proposed device and imaging method is then verified experimentally.
In the following chapter, a coding mask design method is proposed for the aforementioned imaging device. A measurement model is formulatedwhere themask geometry is an explicit parameter to be optimized. After forming this model, a numerical optimization method is proposed and numerically tested. Our numerical experiments show that optimized mask geometries exhibit an energy focusing effect on the region-of-interest, whilst simultaneously decorrelating echo signals between pixels.
In the second part of this thesis, in contrast, we consider methods for calibrating propagation models when the pulse-echo response per pixel is not known. The most important calibration challenge we consider is that of imaging through an aberrating layer in front of an ultrasound array. This could be subcutaneous fat or the human skull, for example. In this thesiswe formulate ameasurement model consisting of a partwhere wave propagation is known (i.e., the assumed homogeneous region behind the aberrating layer, where the contrast image of interest is located), and an unknown propagation part, consisting of the Green’s functions from an array sensor to any point on the the interface of the aberrating layer and the imaging medium. We then investigate methods for finding this set of Green’s functions without explicitly measuring them (so called ‘blind’ calibration).
The first proposed method exploits the singular value decomposition of the measurement data in combination with the assumed Toeplitz structure of the matrices representing the aberrating layer’s Green’s functions. However, the method is lacking in practicality since an additional set ofmeasurements is required with a phase screen mounted on the interface of the aberration layer and the imaging medium. The second method resolves these practical issues by utilizing a covariance matching technique. A sufficiently large set of measurements is obtained where each measurement is different due to e.g. moving particles such as blood flow or micro-bubbles. Using the covariance of the data, algorithms are then defined that can estimate the transfer functions of the aberrating layer from the measurement covariance data.
Finally, we propose a method for estimating the electro-mechanical impulse response of an ultrasound sensor, by simply measuring its pulse-echo response from a flat plate reflector in front of the sensor. Estimating the one-way (electro-mechanical) impulse response then becomes a de-autoconvolution problem, for which we propose a method by solving a semi-definite relaxation of the de-autoconvolution problem.
Additional information ...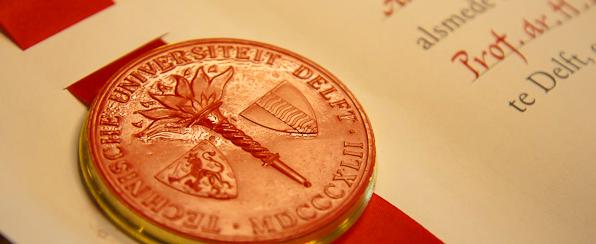